Scientific Report 967
This report is also available as a PDF document .
Abstract
Desert ants forage individually and without the use of chemical trails, yet can efficiently relocate their nest or a food source over long distances in barren or complex environments. Thus, they are an inspiration to engineers wishing to develop robust yet parsimonious navigational algorithms, and neuroscientists seeking to understand how tiny brains can perform such seemingly complex tasks. A range of navigation mechanisms have been hypothesised, but systematic models shown to account for and predict the ant’s behaviour are still lacking. This can be traced to two gaps in current knowledge: a log of the exact movements of the animal leading to the development of a known route, and an accurate reconstruction of the sensory information perceived throughout. Coupling such data with the known optics of the ant eye would allow researchers to reconstruct the entire visual history of the animal - the first such data-set for any animal – providing a gold standard test-bed for those seeking to understand the insect brain or develop autonomous navigation systems.
In this study we sought to address both identified issues by firstly tracking individual desert ants as they traversed their natural surroundings, and then performing a high precision mapping of their visually rich habitat. Custom recording equipment was developed to augment three Leica DGPS units secured through a GEF equipment loan allowing the position and orientation of individual ants to be observed. In a separate collaboration the animal habitat was mapped using 3D laser mapping techniques allowing the sensory input experienced by the animal to be recovered.
Background
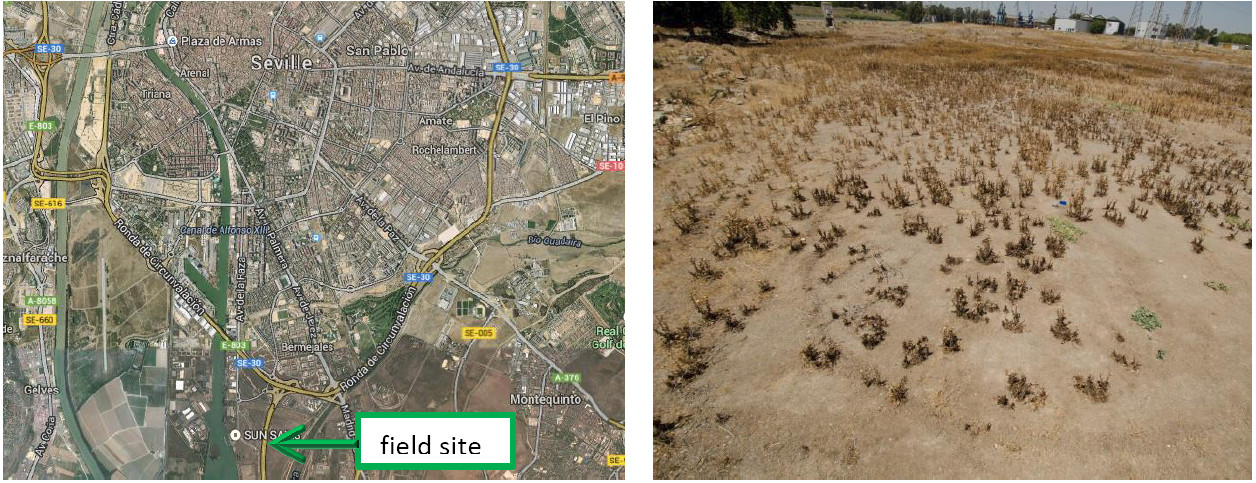
A field study was conducted in the summer of 2012 in wasteland south of Seville, Spain (see figure 1). This site provided various nests of the chosen ant species (Cataglyphis velox) in a flat, secure and visually diverse habitat ideal for our study. The primary aim of the tracking section of this work was to record the position and orientation of individually tracked ants as they searched for food in their natural environment. Indeed, our goal was to record the paths taken by individual ants across their entire foraging lives. That is, from the first time each ant exited the nest, through route learning to a regular feeding site and ending after experimental testing. This is essential to provide accurate training data to models seeking to replicate insect learning.
To the best of the author’s knowledge there is no ‘off the shelf’ solution that satisfies these needs and thus with the aid of the GEF team and Informatics technicians we augmented the standard differential GPS system secured with a GEF equipment loan to record the additional data required.
Augmented DGPS system
To reconstruct the visual input experienced by ants it was crucial to not only track the position of the animal but also its body orientation. Thus, a custom backpack was developed that consisted two DGPS units (Leica GX1230), a high definition wide-angle camcorder (Panasonic HDC-TM900), and an inertial motion unit (Proto-pic razor IMU) as shown in figure 2. The use of the horizontal boom allowed the experimenter to track the ant without disturbing her by maintaining physical separation. The task for the experimenter was therefore to maintain the position of the camcorder directly above the ant being tracked. This was made easier by mounting 4 downward facing laser pointers around the camcorder. Thus the task of the experimenter was simplified to keeping the target ant within the 4 laser dots projected onto the ground. The primary DGPS unit antenna was mounted directly above the camcorder, and thus gives an accurate 3D position of the camera. The second DGPS antenna was mounted at the base of the boom arm, above the shoulder of the experimenter. Combining the position of both GPS antennae allows the orientation of the boom arm to the deduced and thus orientation of the camera. This was necessary to transform the ant body orientation from pixel space in the video to real world space. Real-time kinematic data (RTK) was sampled at 2Hz, using a custom profile implemented by Colin Kay.
We also sought to measure the orientation, roll, pitch and yaw of the camera using a 9 degree of freedom inertial motion unit. The device itself was mounted at the base of the camcorder, in a custom housing with a customs power and level shifter board developed in house by the Informatics technicians. Colin Kay of the GEF team developed a specific profile to read from the IMU and initial testing showed positive results. However, field testing revealed that the Leica data recorder could not store data from 3rd party devices at our sampling rate of 2Hz. It was decided that reducing the sampling rate was not desirable and thus the IMU was unused in our study.
Survey procedure
Tracking of ants was performed by a team of two experimenters. Ants were individually marked with a colour code using enamel paint allowing each to be identified. The “ant-handler” was responsible for identifying ants as they exited the nest, and then manually aiding the ant’s escape from the artificial barrier surrounding the nest. Once released the second experimenter would follow the ant, keeping the camera above the individual. On returning to the nest ants were able to return without aid.
Air temperatures during July, 2012 were often in excess of 40 degrees Celsius with surface temperatures proportionally higher. Thus the ant activity followed a bimodal distribution, with a break in activity in the hottest period of the day. We utilised this window to download all data in the morning and afternoon slots separately and also recharge all batteries.
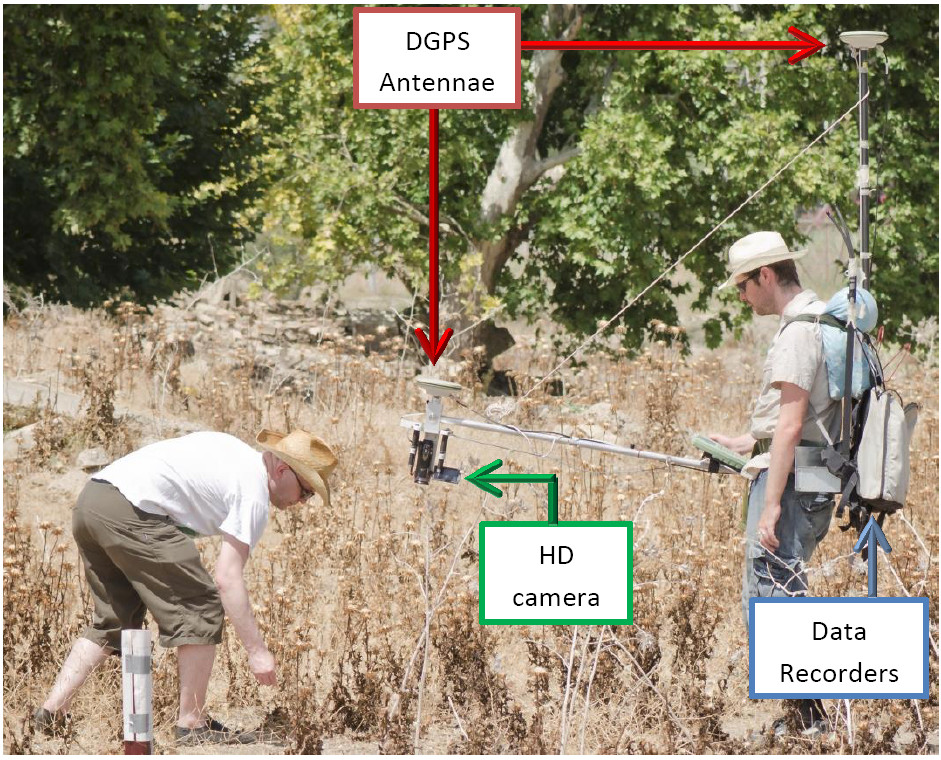
Data gathered
During the field study, the complete foraging lives of more than 18 ants were recorded. Note, that in some cases individual ants were tracked for 3 consecutive days before completing the full experimental test procedure. It should also be noted that the paths of more than 40 ants were recorded but in over half of the cases individuals did not complete the full experimental procedure. The partial data recorded for these individuals shall still prove useful in quantifying the learning behaviours in early foraging journeys. A sample set of routes (the (x,y) position of the primary DGPS unit) are shown in figure 3.
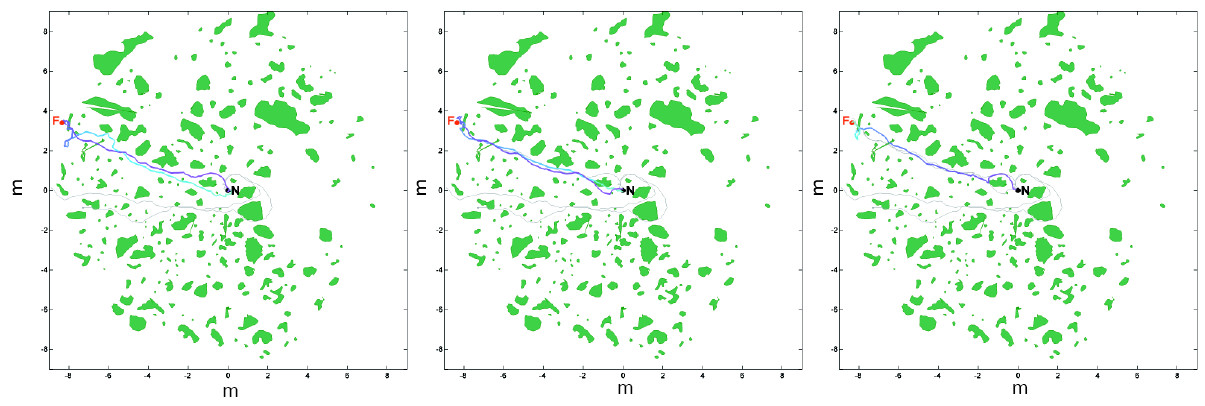
Prior to this study the most common ant tracking method was to lay a grid around the nest area and then manually attempt to plot the position of the animal with respect to the grid on paper or a tablet computer. The accuracy of such methods has never been quantitatively assessed, but it is reasonable to assume that they are fairly imprecise. In contrast the 3D positions of the antennae are accurate to within 2.5cm in over 99% of data points recorded. For the purposes of ant tracking this represents a step change in the resolution of the data gathered precision. The accuracy of ants’ tracks should increase further with tracking the ant in the video sequence. Furthermore, the time required for data entry using the DGPS is significantly reduced in comparison to previous methods.
Interpretation and Preliminary findings
The most immediate result which can be deduced from the routes is that ants can learn a route through their visually dense habitat after a single exposure – known as one-time learning (see figure 3 provides an example). This is noteworthy, as there is considerable data of stereotyped learning behaviours in foraging bees and ants. Thus, there has been an assumption in the field that ant learn routes by foraging in incremental steps using learning walks at key locations. What is clear from our data is that whilst some ants do adhere to such a strategy, other individuals immediately extend their search metres from the nest without performing any learning walks. Amazingly, this does not appear to adversely affect the ability if the animal to relocate its nest naturally or in our experimental manipulation. This result provides a gold-standard test for models of insect learning – they should be capable of navigating the ant habitat after only one pass through. Preliminary data of this type was presented at the Animal Vision Conference, University of Sussex, UK (Mangan & Webb, 2012).
As discussed in the Background section, tracking ants represents the first part of the larger project to reconstruct the visual history of individual desert ants. We are now working to augment the GPS data with the position and orientation of the ant extracted from the video data. Not only should this increase the accuracy of the (x,y) tracking but also should provide the orientation of the animal – something that has never been reported previously. Automatic tracking of the ants in the video data formed the basis of the MSc thesis of Engelman, 2013 (Engelman, 2013) with positive early results. Using an optic-flow based tracking algorithm the position of the ant was automatically extracted from video data with a success rate close to 98% in ideal conditions. We are now working to extend the tracker to work in non-ideal cases (ant in shadow, ant motionless), and also to automatically extract body orientation.
In addition, the mapping of the ant habitat is on-going, which is necessary to complete the data-set. Known issues in 3D mapping must be solved such as extremely large data-sets, failure of foliage to adhere to rigid body constraints for meshing, etc. Once complete, the visual history of each ant shall be reconstructed and published in high impact journals.
The GPS data gathered is also providing a ground-truth for novel video based positioning systems as shown by a second MSc project carried out this summer. Bajerano (Bejarano, 2013) used techniques more commonly used to locate a robot as it navigates to recover the position of the camera in 3D space using only the video data. The DGPS provides ground truth against which to validate results. Again, early results are promising with the algorithms qualitatively approximating the path of the camera in our test cases. Further quantitative analysis is required.
Recommendations
The tracking system discussed in this report represents a proto-type system and as such there are a number of recommendations. As discussed above the IMU was unused as the Leica software would not read from a 3rd party device at 2Hz. However, the data from the IMU is extremely useful and could replace the secondary DGPS antenna carried by the experimenter. Thus, a solution to this issue should be sought before redeployment.
Another issue that has arisen in the data processing is that the video time-stamps are independent of the GPS data. It would be extremely helpful to have video frames accurately synced to the GPS data. It might be possible to develop some additional electronics such that the camera is trigger by the Leica hand-held control unit. In addition, it might be possible to sync the laser pointers to the Leica system, and have the pulsed such that frames could be synced using a pattern of pulses.
Finally, a more comfortable back-pack is a must for future users as carrying the kit for long periods caused back pain for the experimenter. Adapting current back-packs used for carrying heavy garden equipment seems a promising solution.
Conclusions
Desert ants are amongst the most impressive of the animal navigators, searching for food in searing temperatures and recovering their nest quickly despite their small brains and low resolution vision. To date hypothesis of how the animals perform these feats could not be objectively validated as the sensory experience of the animal was unknown. In this work, we used an augmented DGPS system to track individual ants over their foraging lives. The DGPS data gathered is already providing new insights into the learning behaviours of the animals. However, work is on-going to develop novel methods capable of recovering the precise position and orientation of the animals from the video data, and also to reconstruct the ants’ natural habitat.
Publications
Bejarano, D. D. (2013). Tracking Ants in Their Natural Habitat using only Hand-Held Video Data. University of Edinburgh.
Engelman, C. (2013). Tracking Individual Ants in Their Natural Environment. University of Edinburgh.
Mangan, M., & Webb, B. (2012). Reconstructing the entire visual history of individual desert ants. Brighton, UK: Animal Vision Conference
Acknowledgements
This work would not have been possible without the hard work of the GEF team at University of Edinburgh, specifically in providing software support for the Leica equipment prior to deployment and also rapid response to issues occurring in the field. Thanks must also go the technicians in the School of Informatics, University of Edinburgh, for design and construction of the GPS backpack, IMU support electronics, and custom battery packs.