Scientific Report 1071
This report is also available as a PDF document .
Abstract
The original objectives of this loan of 10 6TD seismometers, which supplemented 21 of our own (Cambridge) seismometers were:
- To map patches of deep crustal seismicity (at 12-25 km depth) caused by melt intrusion in the crust in order to constrain models of crustal accretion along volcanic rift zones;
- To monitor the rate of change of induced seismicity around the 2014 Bárðarbunga-Holuhraun intrusion site to constrain rate-state models of earthquake failure following the intrusion;
- To map the ongoing seismicity in the Bárðarbunga caldera and along the Holuhraun dyke path in order to constrain models of dyke cooling and caldera evolution (and possibly eruption).
The deployments and data collection were all successful with a high rate of data return and we are using the data to actively address all three questions, as well as further opportunities that have arisen as a result of having a high-quality long-term dataset from this area of active rifting and volcanism in Iceland.
Background
Over the past decade SEIS-UK has generously supported our work in central Iceland. This has been extremely fruitful both in international publications and in training MSci, MPhil and PhD students. Highlights include recording in detail the 2014-15 Bárðarbunga-Holuhraun dyke intrusion; a detailed tomographic velocity model around the active volcano of Askja; mapping for the first time tiny repeating earthquakes in the deep crust on the Askja rift caused by melt migrating up from the mantle; mapping pervasive strike-slip faulting accommodating rifting on the northern rift zone; showing that the static stress change caused by the dyke intrusion induced an increase of seismicity in some areas and a complete shutdown in others at stress drops of only a few 100 kPa; and making an Icelandwide tomographic model of the top 15 km of crust from ambient noise analysis.
There is huge value in having long-term recording, which has shown its importance in our recent ability to tease out annual seasonal changes in velocities of a fraction of one percent, and the velocity changes caused by the stress changes created by the 2014 dyke intrusion (Donaldson et al., 2019)[^1]. This loan of 10 SEIS-UK instruments has enabled us to maintain recording at important sites over a period of more than a decade, as well as to reposition parts of our array to monitor the ongoing inflation of Bárðarbunga caldera. SEIS-UK provided the instruments, cabling and solar panels, while Cambridge provided the infrastructure for each site (batteries, solar panel stands, vaults).
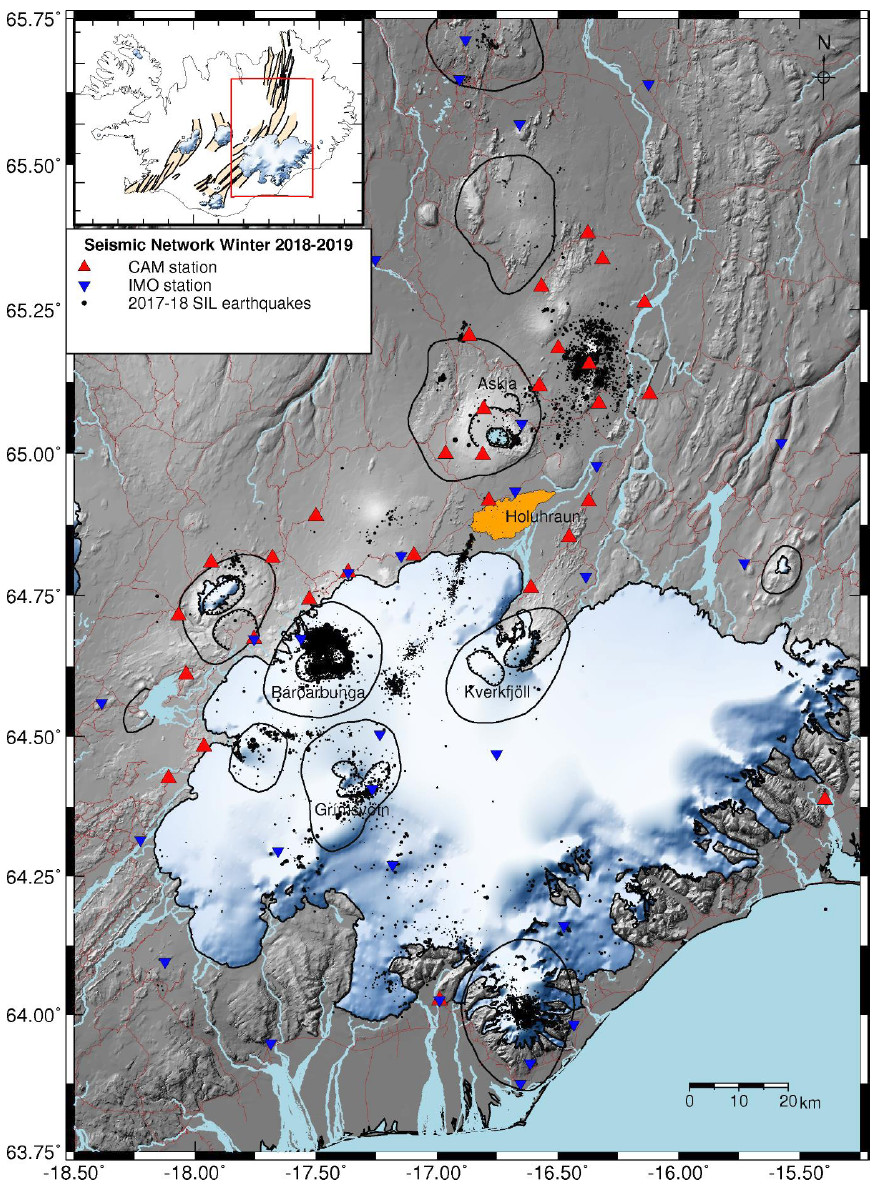
Survey procedure
The seismic data from 2017-19 was recorded continuously at 50 sps, with GPS always on. This was chosen rather than the more normal 100 sps because 16GB instruments would fill up in c. 10.5 months, and we are only able to service instruments once per year in the summer. It was a fortuitous change because COVID-19 restrictions in 2020 meant that the instruments were recording for over 13 months before we were able to service them in August 2020. No data was lost due to storage restrictions.
Our experience is that almost continuous daylight in summer (this is near the Arctic circle), together with the use of large truck batteries and multiple solar panels on each site, provides sufficient power supply through both the summer and winter months for 6TD seismometers. Typically we use three to four 115 Amp-hr batteries with minimum 60 watts solar panels on each site. Solar panels are mounted subvertically to reduce snow adherence in the wetter spring months and to catch the low angle returning sun (Fig. 2). The GPS antenna is attached to the top of the stand so that it projects above the snow cover in winter. We bury the batteries and solar panel regulators, but mount the breakout box on a short stick: this is because when they are buried, they are more prone to becoming flooded as the snow melts. It is also easier to access the sockets for servicing. Our experience of wind turbines is that they do not survive the harsh winter conditions and also produce excess noise, polluting the seismic recordings (Fig. 2).
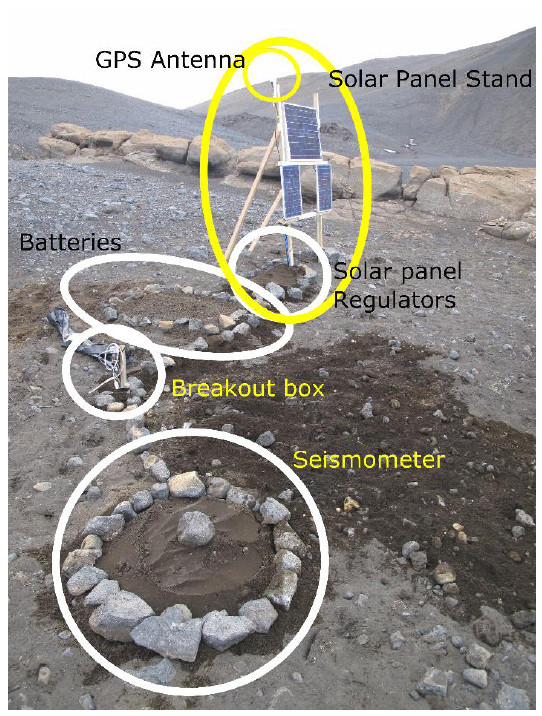
The prevalence of basaltic rocks means that compasses are unreliable indicators of true north, so we use GPS to orient the seismometers. It is best to use a differential GPS receiver, but when that was not available we found that a normal hand-held GPS unit could be used equally well. We took a fix at the seismometer and then walked quickly 100-200 metres either north or south (depending on the terrain) and erected a pole at that point to provide a visual pointer for aligning the seismometer. At these high latitudes there is excellent satellite coverage, and provided little time was spent in locating the position for the pointer (by simply keeping the longitude on the GPS the same as you walked), the normal time-varying positional errors in the GPS were minimised to less than the practical accuracy of aligning the seismometer.
For a few of our sites we make vaults by burying and concreting fish barrels. We have experimented with packing insulation around the seismometer inside the fish barrel, but have found that it is generally deleterious to the signal because if it gets wet and waterlogged it makes the recordings much worse than if any water ingress is simply allowed to drain away. But we find that simply burying the seismometers, especially where there is hyaloclastite outcrop, produces excellent results. In winter the seismometers freeze into the ground (typical temperatures go down as low as -14C), and couple extremely well. The layer of snow and absence of vegetation or trees reduces wind shear and together with the absence of cultural noise provides a very low noise environment. We recorded events typically down to magnitude -0.5 in the upper crust and -0.2 even at 20 km depth.
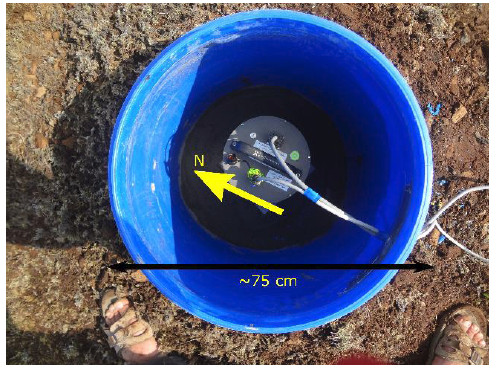
The most difficult periods are during the spring thaw, when we have sometimes seen seismometers tilting as the ground thaws. On Guralp 6TDs we switch off the auto-centring because once the sensor is up against the end stops it causes frequent spikes across all the channels with repeated failed attempts to auto-centre it. In extremis we would rather lose a horizontal channel and keep the vertical sensor (which is less sensitive to tilt) operating without such noise spikes. We have recently suffered from several failures of regulators, due we think to water ingress to the fuse, which is not encased in protective gel filling.
During the deployment period we also suffered from the GPS WNRO wrap-round problem. SEIS-UK were able to supply us with GPS units that lasted beyond the 2019 crunch point, although we then had to change some of them again in 2020 to avoid further WNRO problems. No data was lost due to WNRO issues.
Processing and modelling
After initial quality control checks the data was converted to miniSEED format. Earthquakes were detected and located using our home-grown Continuous Microseismic Mapping (CMM) algorithm (Drew, White et al. 2013 Coalescence Microseismic Mapping, Geophysical Journal International, 195, 17731785, doi: 10.1093/gji/ggt331). This searches in time and space using a signal onset (STA/LTA short time averaging vs. long time averaging) function for event detection and initial location. At the time of writing this is being replaced by the more versatile QuakeMigrate algorithm, written in Python (Winder, T. et al., in prep. and ESSOAr, 2021), which is available for public use from GitHub https://github.com/QuakeMigrate/QuakeMigrate (see example output in Figure 4 below).
Initial hypocentre locations were refined using NonLinLoc and HypoDD for relative relocations. Locations of events are refined by using waveform coherence to pick relative phase arrival times, with accurate relative locations calculated for clusters of events. Moment-tensor solutions are made using interactive software developed in-house which uses the polarities and amplitudes of both the compressional wave arrivals and the radial and transverse shear arrivals in a full Bayesian inversion (Pugh, White & Christie 2016: A Bayesian method for microseismic source inversion, Geophysical Journal International, doi: 10.1093/gji/ggw186). The way in which stresses generated by the 2014 melt intrusion and the Bárðarbunga caldera collapse trigger brittle failure in the overlying crust is being modelled with programs such as Coulomb-3 following our successful application of these methods to the nearby 2007 Upptyppingar intrusion and the 2014 Holuhraun intrusion.
Data quality (including example data)
Data quality (including example data) The data quality is very good: we are able to use it not just for microseismic mapping, but also for receiver function analysis, particle motion analysis for anisotropy and ambient noise studies. Even in the harsh conditions they experience through the winter, we are achieving typically 95% data recovery. An example of data and the automatic location using QuakeMigrate from a single event at a depth of 4.2 km with a local magnitude of 0.06 is shown in Fig. 4 below.
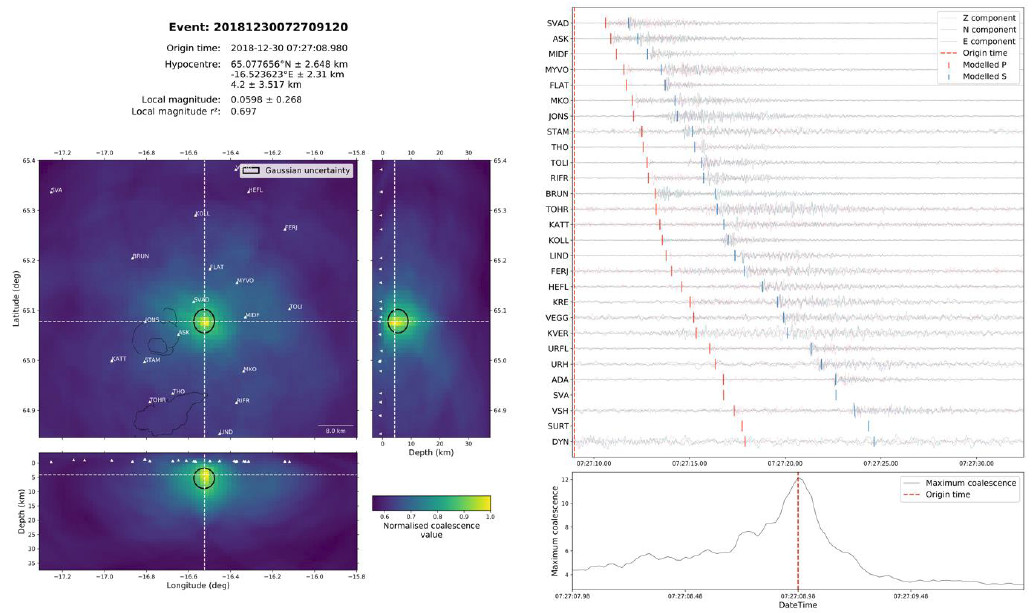
Preliminary findings
-
We have mapped new patches of deep (1525 km below sea level) seismicity at several places along the rift and it is clear that these are of importance in the way melt is fed into, and forms the crust in a way that has been little recognised in the past (most focus has hitherto been on the high-level magma chambers and mush zones in the upper part of the crust). The deep activity near Bárðarbunga appears to be increasing since the end of the eruption, signifying an important melt feeder from the mantle to the crust.
-
We continue to monitor the rate of change of induced seismicity around the 2014 Bárðarbunga-Holuhraun intrusion site to constrain rate-state models of earthquake failure following the intrusion. This is a long-term project: sensible estimates of crustal viscosity and relaxation suggest it will take on the order of a decade for shallow stresses to relax, and we are now approaching that time period since the intrusion. Meantime, every year allows us to add better constraints to the modelling.
-
Seismicity is ongoing in the Bárðarbunga caldera with GPS suggesting it is reinflating. Our moment tensor solutions suggest that normal faults generated during the caldera collapse in 2014 are now reversing their motion in the same locations and are being reactivated as reverse faults.
In addition we are using the data for the following studies:
-
Mapping anisotropy in the crust of this region (in brief, it is fast along strike of the rift and dykes in the shallow crust, and perpendicular to that in the deeper crust where the fast direction aligns with the spreading direction, likely due to shearing and alignment of minerals).
-
We are starting a project to map the attenuation structure of the rift zones and central volcanoes within it, which should help constrain the location of melt or mush zones in the crust. Conclusions and recommendations
The SEIS-UK instruments have been invaluable for supplementing our own Cambridge seismometers, and have enabled us to maintain long-term monitoring of the seismic activity in this active rift zone. The SEIS-UK staff have provided outstanding support throughout, including Victoria Lane travelling to the field with us when Guralp firmware upgrades were required to correct an error in their former software which could cause the seismometer to lock up and stop recording when the memory was full. These upgrades had to be done in the field with only a 15 sec window to complete a critical stage before the seismometer locked up. SEIS-UK also helped us navigate the unexpected problems caused by WNRO which only became apparent at short notice. The work would have been impossible without the enthusiastic support and help of Bryndís Brandsdóttir and Sveinbjörn Steinthórssen of the Science Institute, University of Iceland. We also thank Heidi Soosalu and many students who have helped with the fieldwork. We are pleased that Prof Nick Rawlinson at Cambridge University has joined the Iceland team and is supervising new Masters and PhD students on the data: the world-class dataset is likely to continue to provide new results and understanding of the tectonics and magmatism of this active area, as well as the seismometer array sitting ready to record the next volcano-tectonic crisis, which is likely to be imminent.
It would certainly be helpful if SEIS-UK were to purchase a GPS North pointer instrument because on magnetic basaltic rocks it is impossible to use a magnetic compass: I note that this would also be useful in deployments elsewhere because one of the most common errors in orientating seismometers is applying the magnetic declination in the wrong direction, which is not immediately obvious, but then makes anisotropy measurements unreliable.
Location of the archived data
The raw and miniseed data are archived at Bullard Laboratories, Cambridge University on two different RAID arrays in different buildings, and also at SEIS-UK. The data (together with data from Cambridge seismometers) will be uploaded to IRIS 3 years after the end of the loan when the current PhD students have finished their analysis of the data, together with all the data from our own seismometer stations (as listed in the Table). In the meantime, we are collaborating and providing this data for research with several researchers in the UK and other countries (Oxford, USA, Germany, Belgium, Estonia, Iceland and France).
The locations of all the microearthquakes we have identified are published in Supplementary Information of our publications, where they are publicly available for download.
Refereed publications
Donaldson, C., Winder, T., Caudron, C. & White, R. S. (2019). Crustal seismic velocity responds to a magmatic intrusion and seasonal loading in Iceland’s Northern Volcanic Zone, Science Advances, 5, eaax6642
Volk, O., White, R. S., Pilia S., Green, R. G., Maclennan, J., & Rawlinson, N. Oceanic crustal flow in Iceland observed using seismic anisotropy, Geophysical Journal International (in review)
Volk, O., White, R. S., Greenfield, T., Bacon, C., Winder, T., Rawlinson, N. Crack-induced seismic anisotropy dominates shallow crustal velocity structure beneath Askja volcano, Iceland: Implications for body and surface wave models, Geophysical Research Letters (in review)
Winder, T., Bacon, C. Smith, J. D., Hudson, T. S., Drew, J. and White, R. S., QuakeMigrate - a Python package for automatic earthquake detection and location using waveform migration and stacking, Seismological Research Letters, in prep. and at Earth and Space Science Open Archive (ESSOAr), https://doi.org/10.1002/essoar.10505850.1
White, R. S., Edmonds, M., Maclennan, J., Greenfield, T. & Ágústsdóttir, T. (2018). Melt movement through the Icelandic crust, Proceedings of the Royal Society, Series A, 377, 20180010, doi: 10.1098/rsta.2018.0010
White, Robert (2020). Fire and fury in Iceland: tracking volcanic eruptions, Transactions of the Leicester Literary & Philosophical Society, 114, 1922.
PhD dissertations using data from SEIS-UK instruments
2019 Clare Donaldson: Towards monitoring volcanoes in Iceland and Hawai’i with seismic velocity variations
2020 Omry Volk: Ambient noise constraints on Icelandic crustal structure
(2021) Tom Winder: Tectonics of the northern volcanic zone, Iceland from automatic detection and location of microseismicity
(2021) Conor Bacon: Seismic anisotropy of Icelandic crust
Conference Abstracts
Clare Donaldson, Robert S White, Corentin Caudron (2018), Annual seasonal variations in relative seismic velocity changes in the Northern Volcanic Zone, Iceland, Geophysical Research Abstracts, 20, EGU2018-15393
Conor Bacon et al. (2018) Seismic anisotropy in the Icelandic rift zone, Geophysical Research Abstracts, 20, EGU2018-13641.
Winder, T. et al. (2018) A Surge in Seismicity in a Network of Cross-Cutting Conjugate Strike-Slip Faults Triggered by the 2014 Bárðarbunga-Holuhraun Dike Intrusion, American Geophysical Union, Fall Meeting, Abstract
Conor Bacon, Robert S. White, Nicholas Rawlinson (2018) Seismic anisotropy in the Icelandic rift zone, Postgraduate Research in Progress
Brandsdóttir, B., White, R. S., Ágústsdóttir, T., Winder, T., Smith, J. D. & Bacon, C. (2019). Caldera Seismicity Associated with the 1998 Inflation of the Shallow Crustal Magma Chamber beneath the Grímsvötn Hotspot Volcano, Iceland, American Geophysical Union Fall Meeting, 2019
Conor Bacon, Robert S. White, Nick Rawlinson (2019). Depth Constraints on Seismic Anisotropy in Iceland from Shear Wave Splitting Measurements, British Seismological Meeting, 2019
Conor Bacon, Robert S. White, Nick Rawlinson (2019). Depth Constraints on Seismic Anisotropy in Iceland from Shear Wave Splitting Measurements, American Geophysical Union Fall Meeting, 2019
Omry Volk, Robert S White, Simone Pilia, Robert G Green, John Maclennan & Nicholas Rawlinson, (2019). Crustal Flow and Formation in Iceland from Radial Anisotropy, American Geophysical Union Fall Meeting, 2019
White, R.S., Woods, J., Winder, T., & Brandsdóttir, B., Agustsdottir, T. & Hudson, T. (2019). Tracking subsurface melt movement through the crust in Iceland using seismology, American Geophysical Union Fall Meeting, 2019
Tom Winder, Clare Donaldson, Robert S. White (2019). Seasonal Seismicity and Seismic Velocity Variations across the Northern Volcanic Zone, Iceland, are Controlled by PorePressure Variations and Elastic Loading, American Geophysical Union Fall Meeting, 2019
Clare Donaldson, Tom Winder, Corentin Caudron, Robert S. White (2019). Crustal Seismic Velocity Responds to a Magmatic Intrusion and Seasonal Loading in Iceland’s Northern Volcanic Zone, American Geophysical Union Fall Meeting, 2019
Southern, E.O., Winder, T.E.B. & White, R.S. (2020). Bárðarbunga Caldera Collapse and Re-inflation in Iceland During 2014-2019, VMSG Plymouth January 2020.
Winder, Tom & White, Bob (2020). Slowly migrating tectonic microearthquake swarms in the Icelandic Rift Zone: driven by fluid migration or aseismic slip transients? Geophysical Research Abstracts, Vol. 22, EGU2020-19827, EGU General Assembly 2020.
Tom Winder, Conor Andrew Bacon, Jonathan Daniel Smith, Thomas Hudson, Tim Greenfield and Robert S White (2020) QuakeMigrate: a Modular, Open-Source Python Package for Automatic Earthquake Detection and Location, American Geophysical Union Fall Meeting, 2020
Esme Southern, Tom Winder and Robert S White (2020) Insights from an analogous eruption: Bárðarbunga caldera collapse and re-inflation in Iceland during 2014-2019, American Geophysical Union Fall Meeting, 2020, Abstract ID# 741469
Table of instrument deployment details during summer 2018 to summer 2019
