Scientific Report 1008
This report is also available as a PDF document .
Abstract
Ground penetrating radar (GPR) data were collected on the forefield of Isfallsglaciären, Tarfala, northern Sweden. The aim of the survey was to gather information regarding the internal composition of flutes, a type of subglacial bedform commonly found on glacier forefields, the origin of which is debated. Adverse weather conditions, combined with the clayey, boulder-rich substrate that was surveyed limited the utility of the GPR. Future work will focus upon characterising the morphology of the forefield’s flutes, which were imaged using a UAV during the field campaign.
Background (including field survey site location map)
Elucidating the processes which occur at the base of glaciers is key to understanding how they move over their beds (Weertman, 1957), and is therefore important for assessing the response of glaciers to climatic perturbations (Alley and Jougin, 2012). Emphasis on subglacial bedforms has grown since the realisations that subglacial sediments can regulate glacial motion (Boulton and Jones, 1979), bedform morphometry could reflect ice flow conditions (Stokes et al., 2013), and bedforms contribute to basal drag. Flutes are a subglacial bedform commonly found in many recently exposed glacial terrains (Figure 1). They are elongate ridges of glacial sediment that typically develop from the lee side of a boulder, and extend parallel to the ice flow for some tens to hundreds of metres. Despite their relatively limited size, flutes appear to have many similarities with other, larger subglacial landforms (for example drumlins or mega scale glacial lineations). Thus, flutes may belong to a much larger continuum of bedforms that might share a common, currently elusive, genesis (Rose, 1987). Therefore, improved understanding flute formation will help constrain the dynamic interaction between the ice and its bed and might also shed light on the formation of other bedforms. However, there is no consensus on how flutes form.
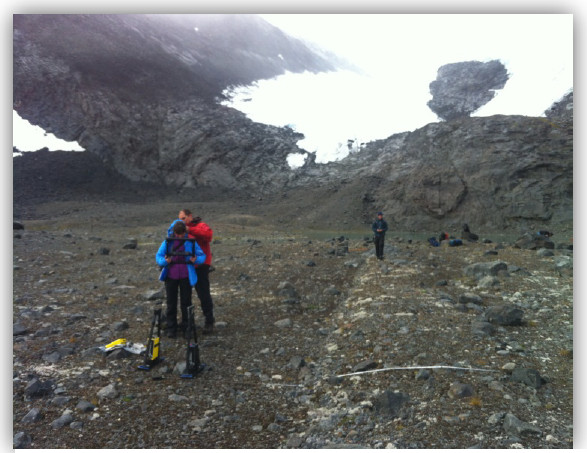
The favoured model of flute formation regards them as the infill of deforming subglacial sediment into a propagating subglacial cavity formed in the lee-side of a boulder (e.g. Boulton, 1976). In this scenario, the development of flutes may reduce the amount of sediment available for subglacial deformation, regulating ice velocity and acting as “sticky spots” in a mosaic of stable and deforming bed conditions (Piotrowski et al., 2004).
Alternatives to the cavity infill model have been proposed, based upon observations of flutes without a stoss-side boulder and flutes that are regularly spaced (Baranowski, 1970; Gordon et al., 1992; Schoof and Clarke, 2008).The frost heave hypothesis (Baranowski, 1970) builds on the cavity infill model by producing regular obstacles to ice flow in the form of frozen sediment. This regular freezing is caused by frost heave from a permafrost layer, situated underneath the cold based region of a polythermal glacier. Conversely, the spiral flow hypothesis (Schoof and Clark, 2008) requires no basal obstructions. Instead, flutes are generated via an instability mechanism whereby spiral flows in basal ice concentrate sediment between adjacent spirals. This hypothesis relies on a weak, Reiner-Rivlin rheology for basal ice. All hypotheses proposed so far imply different initial subglacial conditions and ice-bed interactions, and it is therefore vital to identify which one is correct.
During the week-long field work at Tarfala (Sweden) we used ground penetrating radar (GPR), accompanied with sedimentological field measurements, to study flute internal composition in the recently deglaciated forefield of Isfallsglaci ren (Figure 2). Particular emphasis was put in the identification of (i) the diamicton-bedrock boundary below the landforms and (ii) any structure within the diamicton. The GPR analysis was only one of several techniques used in the investigation of these landforms during the field work, including clast fabric analysis and the creation of UAV derived high resolution DTMs to study flute morphometry.
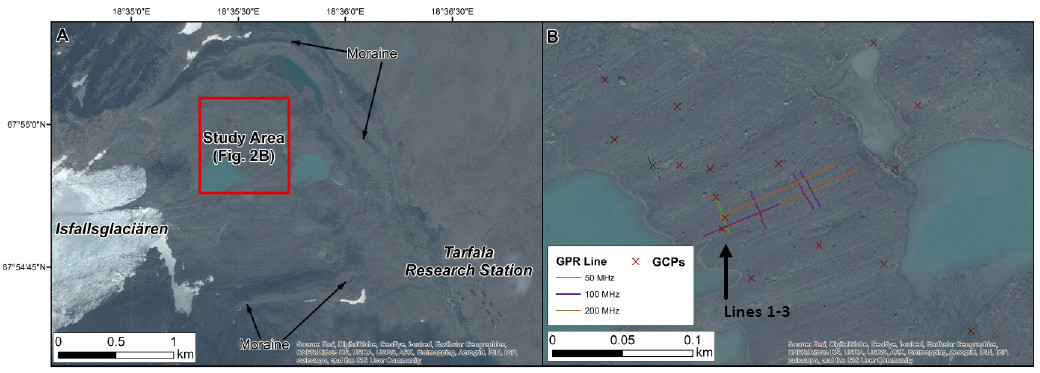
Survey procedure
The internal characteristics of several tens of flutes were imaged with 200, 100 and 50 MHz GPR antenna, connected to a dGPS. Sampling strategy included acquisition of intersecting radargrams both along and across the landforms. The location of the GPR lines is shown in Figure 2B and details are provided in Table 1. Radar lines were often diverted due to obstacles such as boulders on the surface of the study area (e.g. Figure 3). The dGPS was also used to collect several GCPs for photogrammetric surveying, which were distributed throughout the study area (Figure 2B).
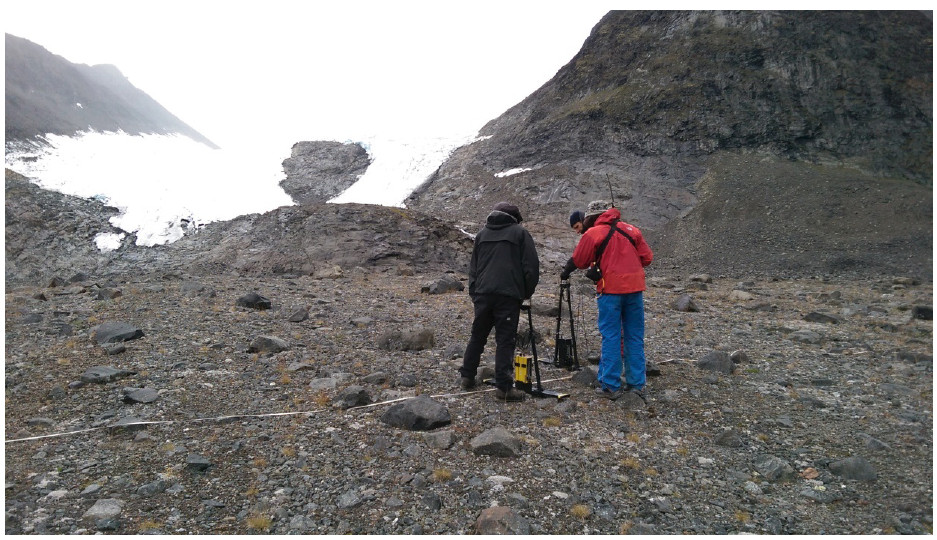
Data Quality
The quality of data collected was relatively good, i.e. the antennas worked well, despite the harsh conditions (sub zero temperatures etc.). However, the clay-rich diamiction made it extremely hard to penetrate beyond the shallowest carapace of the landforms, while the adverse weather conditions (it was almost constantly raining) forced us to limit our total acquisitions to 15 lines. Repeat surveying showed the 200 Mhz antennae to be potentially the most useful (Figure 5). As this conclusion was reached in the field, the majority of lines collected used the 200 Mhz antennae (Table 1).
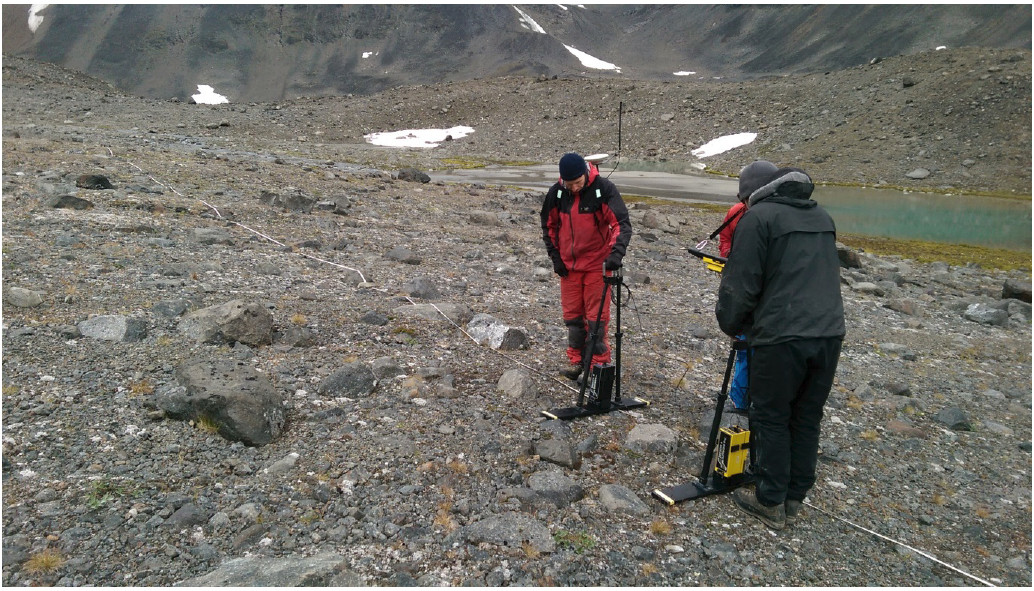
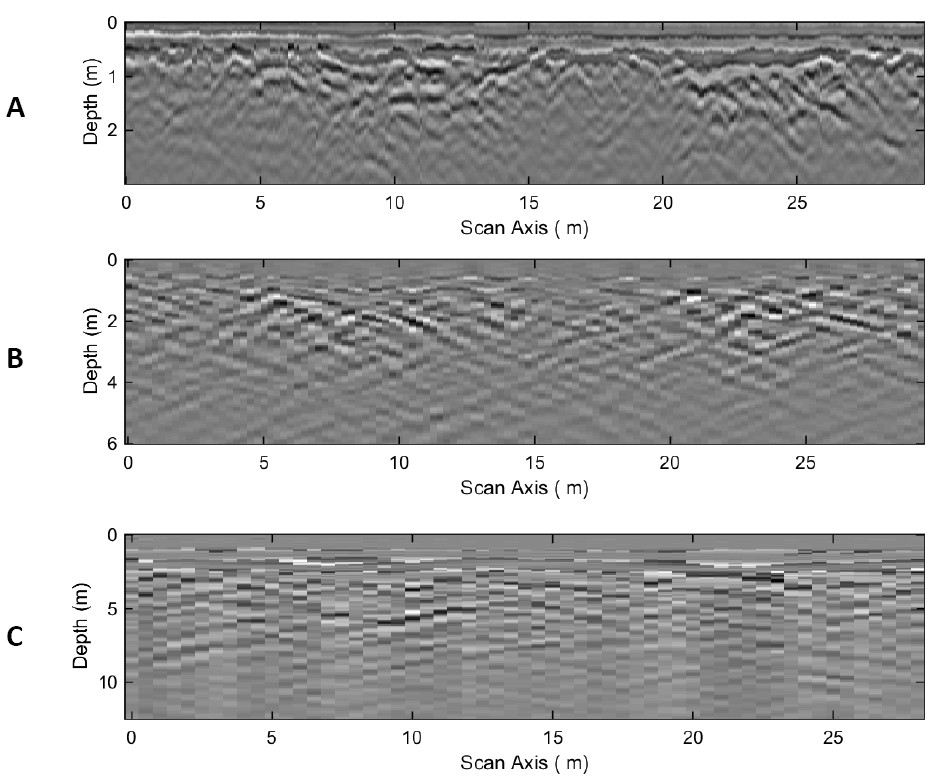
Processing and modelling
Initial processing of the GPR data (e.g. Figure 5) was undertaken using the procedure outlined in Spagnolo et al. (2014), using matGPR (Tzansis, 2010). Specifically, the following workflow was applied:
- The signal position was adjusted to remove the data recorded prior to the wave penetrating the sub-surface.
- A global noise removal, determined from the mean trace, was applied to remove system noise.
- A low pass frequency filter and a high pass wavelength filter were applied to supress noise.
- Inverse amplitude and inverse power gain corrections were made in order to compensate for signal attenuation.
- The time window was `trimmed’ to remove late-time arrivals beyond the penetration depth.
- A 1-D time-depth conversion was made, assuming a constant velocity of 0.1 m/ns.
Full-post processing has yet to be completed, as the initial investigation of the GPR data suggested they reveal relatively little information, therefore making the morphometric analysis the current priority in the post field trip research.
Interpretation to date
The preliminary interpretation of the acquired radargrams, so far, indicates that the GPR was unable to penetrate through the diamicton to the bedrock, with the exceptions of a few cases where this was extremely close to the surface (almost visible). The internal composition of the flutes is largely structureless, although it is not entirely clear what depth of penetration was reached. It also demonstrates that flutes are not just well defined ridges superimposed on sediment of different nature, but most likely represent the positive expression of a continuous, waveform surface. However, this argument also requires knowledge of the penetration depth.
Preliminary findings
More work is needed in order to fully post-process the acquired data. If the first impression is confirmed, the most important findings in relation to the GPR analysis is the absence of structure in the internal composition of the flutes, and also the lack of evidence of buried boulders in the stoss end of flutes which might have instigated the formation of these landforms.
Conclusions and recommendations
In conclusion, it was very useful to be able to test the use of the GPR in these conditions, although the high clay content and the excessive rain meant that the penetration depth was limited.
Instrument deployment details
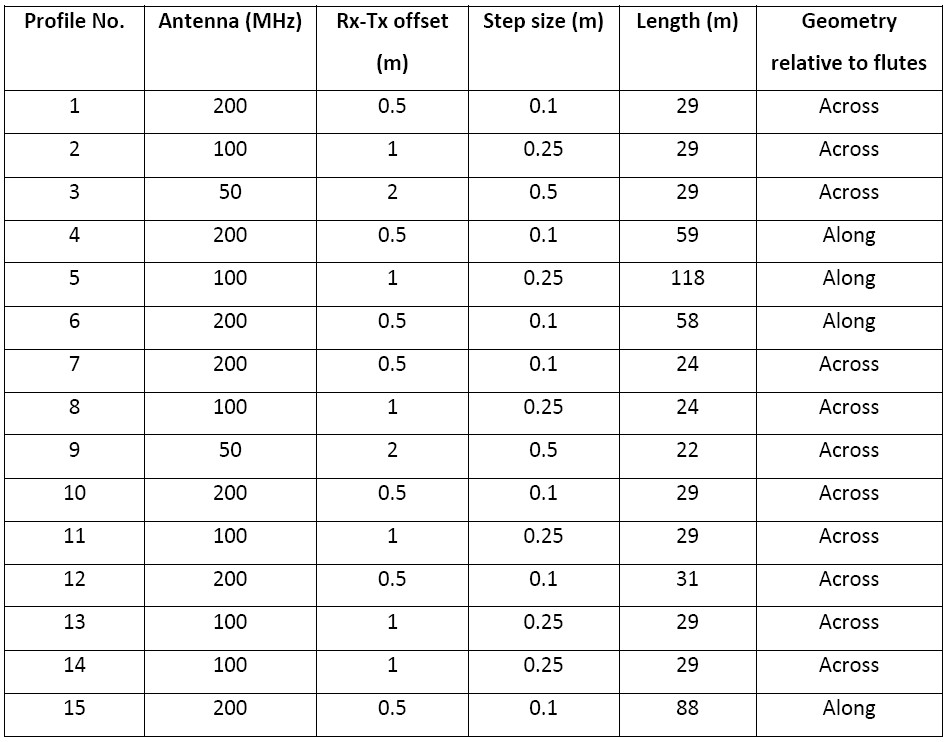
Location of archived data
Data is currently stored in multiple copies in the disk space of the investigators PCs, in Aberdeen, Sheffield and Belfast. Copies of the data can be provided, upon request, by contacting Dr. Matteo Spagnolo, University of Aberdeen (m.spaagnolo@abdn.ac.uk; 01224273034)
Publications, including conference presentations
There has not been any publication or presentation yet on this work, but we will continue working on this project and will forward any relevant publications.
References
Alley, R. B., & Joughin, I. (2012). Modeling ice-sheet flow. Science, 336(6081), 551-552.
Baranowski, S. (1970). The origin of fluted moraine at the fronts of contemporary glaciers. Geografiska Annaler. Series A. Physical Geography, 68-75.
Boulton, G. S. (1976). The origin of glacially fluted surfaces observations and theory.Journal of Glaciology, 17(76).
Boulton, G. S., & Jones, A. S. (1979). Stability of temperate ice caps and ice sheets resting on beds of deformable sediment. Journal of Glaciology, 24, 29-43.
Gordon, J. E., Whalley, W. B., Gellatly, A. F., & Vere, D. M. (1992). The formation of glacial flutes: assessment of models with evidence from Lyngsdalen, North Norway. Quaternary Science Reviews, 11(7), 709-731.
Piotrowski, J. A., Larsen, N. K., & Junge, F. W. (2004). Reflections on soft subglacial beds as a mosaic of deforming and stable spots. Quaternary Science Reviews, 23(9), 9931000.
Rose, J. (1987). Drumlins as part of glacier bedform continuum. In Drumlin symposium (pp. 103-116).
Schoof, C. G., & Clarke, G. K. (2008). A model for spiral flows in basal ice and the formation of subglacial flutes based on a ReinerRivlin rheology for glacial ice. Journal of Geophysical Research: Solid Earth (1978 2012), 113(B5).
Spagnolo, M., King, E. C., Ashmore, D. W., Rea, B. R., Ely, J. C., & Clark, C. D. (2014). Looking through drumlins: testing the application of ground-penetrating radar. Journal of Glaciology, 60(224), 1126-1134.
Stokes, C. R., Spagnolo, M., Clark, C. D., Cofaigh, C. ., Lian, O. B., & Dunstone, R. B. (2013). Formation of mega-scale glacial lineations on the Dubawnt Lake Ice Stream bed: 1. size, shape and spacing from a large remote sensing dataset. Quaternary Science Reviews, 77, 190-209.
Weertman, J. (1957). On the sliding of glaciers. Journal of Glaciology, 3(21), 33-38.